
In an earlier case study last year, we looked at the neutron absorbency of gadolinium and the doors it opens for neutron applications such as contrast tagging in radiographic nondestructive testing. Gadolinium’s extremely high neutron cross section, or its affinity for absorbing and scattering neutron radiation, compared to most other industrial materials makes it a powerful contrast agent in neutron radiography. The higher a material’s cross section, the more opaque it shows up on an image produced by neutron radiography—far more opaque than the surrounding material. For this reason, a liquid gadolinium solution is used to enhance the appearance of hairline cracks and certain foreign materials in neutron images.
Gadolinium is not the only neutron absorber. Boron likewise has an astronomically high neutron cross section, and certain isotopes of boron such as boron-10 have even higher cross sections than their elemental counterpart. This gives boron similar contrast agent properties for neutron imaging, but its neutron absorbent properties also prove useful in other applications of neutron radiation, such as cancer treatment, and in high-radiation environments.
What is Boron?
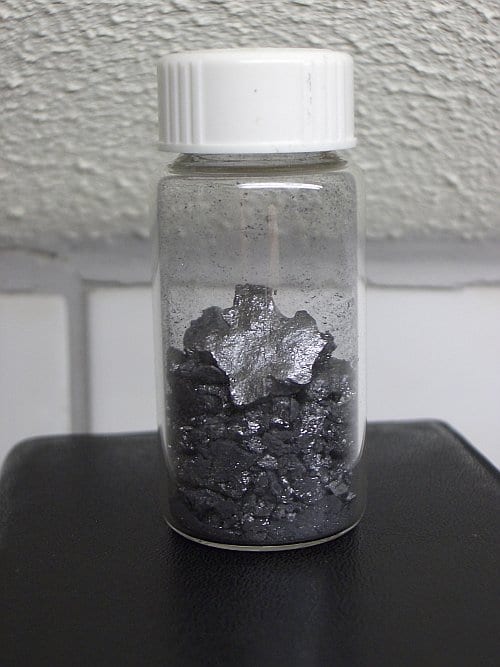
Boron, with an atomic number of 5 (making it the literal fifth element), is a mineral that is not particularly common on Earth—unlike many of the elements comprising the planet’s crust, it wasn’t produced in the stellar nuclear furnace of our sun but rather by cosmic ray spallation and supernovae. Boron is commonly found as part of natural compounds such as borates and borax. It was not discovered as a discrete element until the early 19th century when it was isolated from such compounds.
Most of the world’s boron is used to make boric acid. It is also used to strengthen glass against thermal shock, which is important for laboratory glassware and consumer cookware, and in nuclear reactors as shielding material. Boron compounds are also, as we will see below, found in welding flux.
Boron As Cancer Treatment: Boron Neutron Capture Therapy
Boron’s neutron capture properties makes it a promising therapy for cancer. The process of boron neutron capture therapy, which is not currently widely used but has been undergoing some promising tests, begins with introducing a boron-rich tagging agent into a patient’s body. The boron compounds in the tagging agent bind with tumors within the body. A targeted neutron beam is then shot at the tumor. When a boron atom absorbs a neutron, it becomes excited and releases an alpha particle, a form of radiation consisting of high-energy electrons. Alpha particles kill cells very easily, but do not travel very far, so the neutron-activated boron kills the cancer cells with minimal damage to surrounding healthy tissue.
Boron As a Nuclear Shielding Material
In the world of nuclear shielding and high radiation environments, boron is considered a “neutron poison” due to its cross section. In nuclear reactors, neutron radiation is a large component of fission byproducts. Large amounts of neutron radiation, due to the phenomenon of neutron activation, are undesirable in a reactor—they can weaken or damage structural materials or electronics and can spread more directly harmful forms of radiation, and on top of that they can slip easily through materials such as lead which will block other forms of radiation. Therefore, materials used to shield reactors are often fabricated with a high boron content, especially boron-10. Boron-rich concrete, glass, and other composite materials often see use in radiation-hardened systems.
Boron As a Neutron Imaging Contrast Agent
Boron can be found near other elements such as carbon and nitrogen on the periodic table and shares many physical properties with them, but it does not share its neighbors’ neutron attenuation. Because X-ray attenuation varies roughly linearly with atomic mass, boron and its periodic neighbors have similar X-ray cross sections. Therefore, boron-rich and carbon-rich materials can appear at similar levels of opacity on an X-ray image.

However, neutron attenuation has far more complex rules, and boron has a neutron cross section far, far higher than its neighbors despite having similar mass, which makes it almost as extreme contrast agent for neutron imaging just as gadolinium is, though it has fewer applications.
For example, high-boron-content glassware, which you will often find in a laboratory setting or in your kitchen in the form of Pyrex baking dishes and the like, will reveal itself in a neutron image as being more opaque and foggier than normal glass (which neutrons typically penetrate rather easily).
To demonstrate the N-ray contrast properties of boron, we at Phoenix conducted a little experiment using our neutron imaging system, a copper plate, and two kinds of welding flux.
What Is Welding Flux, and Why Does It Have Boron In It?
As long as humans have been working with metal, flux has been a critical element of metallurgy. Whether in soldering, brazing, smelting, or welding, flux plays an important role. Flux is a spreadable material which is applied to the areas of the base metal that will be part of the welded or brazed joint. Upon heating, the flux melts and reacts with the underlying metal and dissolves surface oxides and other contaminates into a glassy slag that coats the metal and protects the surface from further oxidation. This fluxing action leaves a pristine metal surface on which the filler metal can flow and create a good metallurgical bond.
Without flux, many welding processes wouldn’t work reliably. However, although flux is essential for many types of joining processes, it can also cause problems in a welded or brazed joint. Occasionally a pocket of flux will become trapped within the joint, thereby displacing filler metal and weakening the joint. Additionally, residual flux missed in the cleaning process can accelerate corrosion in that area. For these reasons, residual flux in a joint is not desirable.
Various materials can be used as flux as the situation calls for it. Rosin, for example (as in the same rosin used by violinists to treat their bow strings), is often used as a soldering flux, often as part of a tin-lead compound. For metallurgy stretching back all the way to ancient times, borax was used as flux—borax, as in boron. Borates such as borax and boric acid have numerous uses in welding flux when mixed with other substances, such as lowering the melting point of iron oxide to better remove it from the join.
Neutron Imaging Flux for Contrast
For this experiment, we selected two of the brazing fluxes at our machine shop’s disposal: Superior No. 601 silver brazing paste flux and Superior No. 601B/3411 boron modified silver brazing paste flux for higher temperature applications. The former has a white-silver coloration; the latter is black-silver, and as its name implies, it contains a higher concentration of boron.
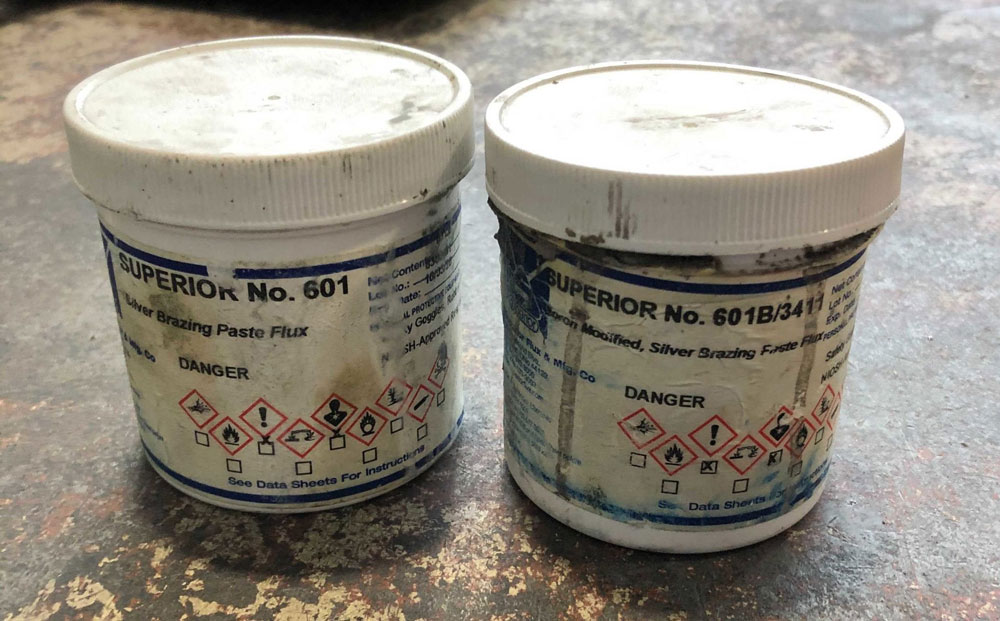
To see if the higher concentration of boron in the boron modified flux will appear on a neutron image, we applied both fluxes to a copper plate (without welding anything), waited for the flux to dry, and ran the copper plate through both our X-ray and N-ray imaging system.

In this photograph of the copper plate, you can see the white flux applied to the right half of the plate and the black boron-modified flux applied to the left half of the plate. The X-ray turns out as one might expect:

Copper, like many metals, is a dense material with a high X-ray cross section, and so it shows up as quite opaque on an X-ray image; boron is a less dense mineral with a lower X-ray cross section, which makes it more transparent. You can increase the energy of the X-rays to more easily penetrate the copper and render it more transparent, but nothing you do to tweak the X-ray output will make the boron appear more opaque than the copper. In other words, no matter what you do, you will never capture the boron-rich flux on an X-ray image.
On the other hand, boron’s neutron cross section is about a hundred times larger than copper’s, so the neutron image ends up looking like this:
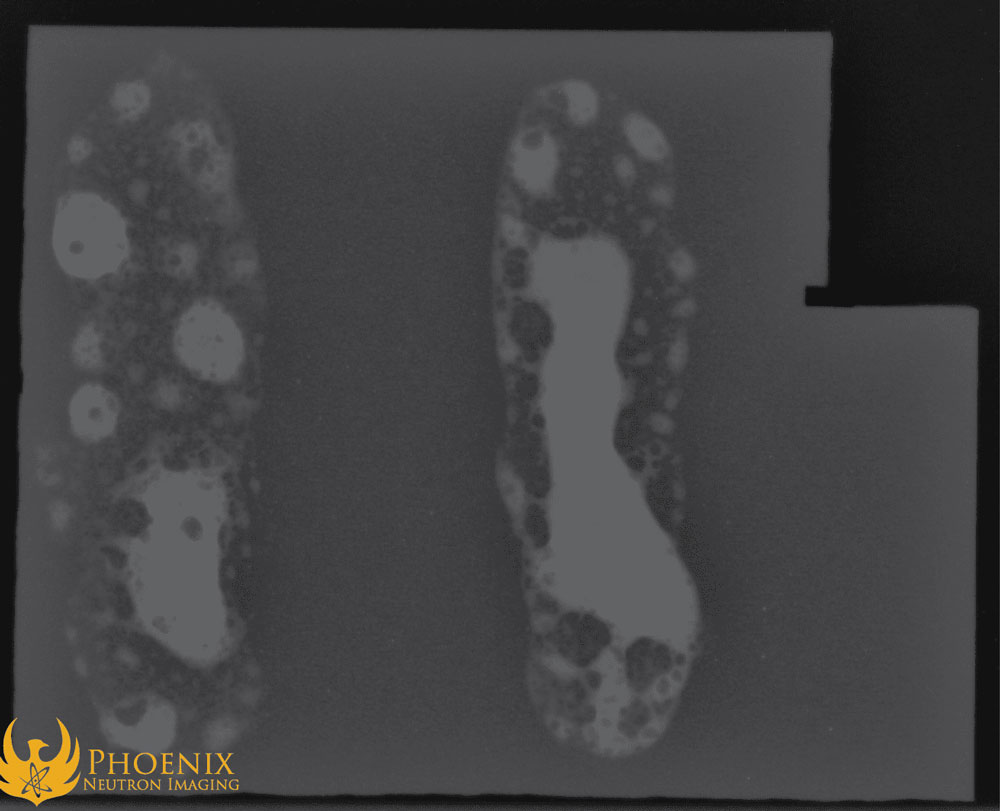
You can see that the blobs of flux are very clearly visible on the neutron image. They would be visible in the image even if they had been buried deep within the surrounding metal! Boron’s neutron absorbent and neutron scattering properties prevent neutron radiation from passing through areas with a high boron concentration, resulting in fewer neutrons making it onto the film.
To our surprise, there appears to be a negligible difference between the two different types of flux as they appear on the neutron images. Our initial expectation was that the black Superior No. 601B/3411 flux might appear as brighter on the neutron image than the white flux due to the presumed increased boron content. However, this doesn’t appear to be the case. It is likely that because both fluxes are dominated by their borax component already, the additional boron in the black flux does not significantly increase the total boron content.
What this result does show is that even relatively small amounts of residual boron-containing flux in a brazed or welded joint would be readily discernible in a neutron image. Since boron is present in many fluxes, neutron radiography can be very effective and powerful as an inspection methodology for brazed and welded joints by detecting some of the problems with fluxes discussed above, such as pockets of flux, which can weaken the joint’s structural integrity, or residual flux left behind after cleaning, which can cause corrosion.